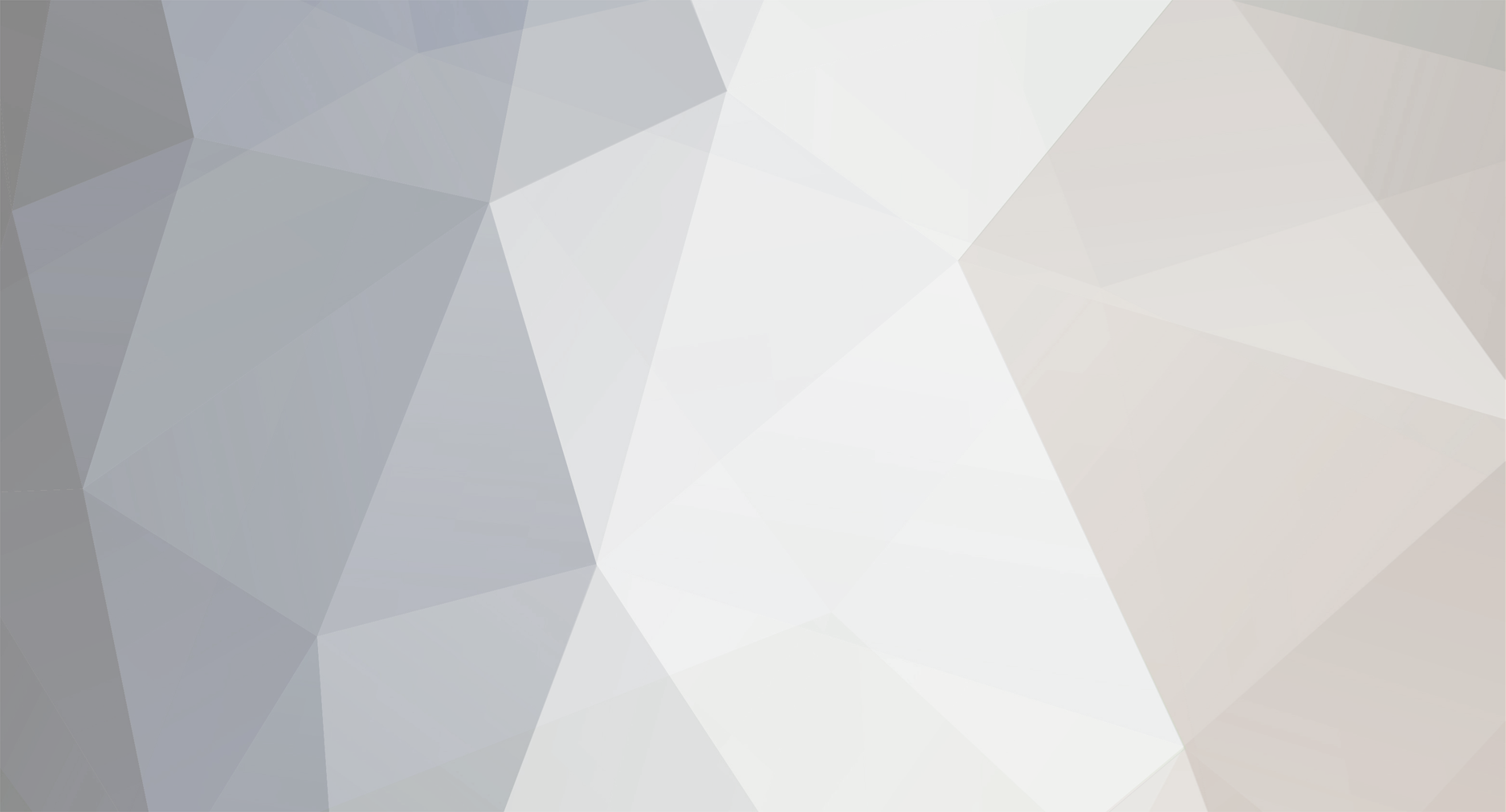
Effigis
Inactive Members-
Posts
2 -
Joined
-
Last visited
Contact Methods
-
Website URL
http://www.effigis.com
Profile Information
-
Gender
Not Telling
-
Location
Canada
-
Interests
GIS - Remote Sensing - Satellite Imagery - Geospatial Technology - Land Management - Geological Studies - Image Interpretation and Processing - GNSS
Effigis's Achievements
Newbie (1/14)
2
Reputation
-
Author: Daniel Macias Valadez, GNSS Analyst at Effigis Original text available here: http://bit.ly/1IYSbNY GPS, being the pioneer of all Global Navigation Satellite Systems (GNSS), is the only system that has remained fully operational for civilian use for more than two decades. The number of civilian applications has exploded in recent years and its popularity is undisputed. Other GNSS have had issues (GLONASS during the end of the 90s and the 00s) or are still incomplete (Galileo, BeiDou). This helps explain the fact that a large percentage of the population is familiar with the term “GPS”, but not so for the other GNSS: GLONASS, Galileo, BeiDou. However, these other systems are expected to catch up in a few years so GPS must maintain its edge and remain competitive. Since the beginning of GPS, technical and performance improvements have been regularly implemented in each new generation (called “Block”) of GPS satellites launched. However, due to the relatively long lifespan of the satellites (between 8 and 20 years), and the fact that satellites are replaced progressively (one or two per year on average), the effects of the performance improvements are gradual and often go unnoticed. During the first generations of GPS, most improvements concerned satellites and the equipment inside them (improvement in clock performance, improvements in satellite autonomy and design, etc.). However, beginning with Block IIR-M, whose first satellite was launched in 2005, new GPS signals have been added. These new signals have several improvements, which, from the user perspective, are interesting because they enable enhanced positioning accuracy and reliability. Before introducing these new signals, let me give you a brief summary of currently used (legacy) GPS signals. Image: Block IIR-M Legacy Signals: L1 C/A, L1P(Y) and L2P(Y) From the beginning, GPS satellites have transmitted three main signals: one civilian signal (L1 C/A), and two encrypted military signals (L1P(Y) and L2P(Y)). As this implies, only one signal is available to civilian users. Military signals are transmitted in two different frequency bands: L1 (1575.42 MHz) and L2 (1227.60 MHz) and are encrypted. Having two bands is highly beneficial in improving accuracy and convergence time, since the undesired ionospheric effects can be greatly reduced. Since the 90s, designers of Geodetic-grade receivers have managed to access the encrypted L2P(Y) signal through a technique called “semi-codeless tracking”, and thus offer all the benefits of having two frequencies to civilian users. However, on the one hand, geodetic-grade receivers have a much higher price tag compared with low-end receivers, and, on the other hand, the techniques used to access the encrypted L2P signal introduce a slight signal degradation. L2C Signal As I previously mentioned, the benefits of receiving a second GPS signal in another frequency band are quite significant. With the ever-growing demand of civilian GPS applications, the U.S. Department of Defense (DoD) decided to include a new civilian signal on the L2 frequency band called L2C, beginning with block IIR-M satellites. Instead of just using a signal identical to legacy L1 C/A and transmitting it on L2, the L2C signal was redesigned to provide several technical improvements when compared with the L1 C/A signal. The L2C uses more sophisticated and modern modulation techniques, which offer the following advantages when compared with the legacy signal: Increased sensitivity or tracking threshold, which translates into an improvement in maintaining the tracking of the signal in unfavourable conditions, such as with obstructions or even indoors. Greater cross-correlation between signals, which enables a stronger tolerance to interference and multipath. Since the L2C is an unencrypted signal intended for civilian use, it is expected that even non-expensive, non-geodetic grade receivers will be able to receive and track the L2C signal. Even though the L2C signal looks promising, we still have to wait a few years before being able to take advantage of it. As of July 2015, out of 30 operational satellites, only 15 transmit L2C. It is expected that all satellites will transmit the L2C signal by 2020. Additionally, even though the first L2C transmitting satellite was launched in 2005, Block IIR satellites began transmitting usable navigation messages (updated daily) on December 31, 2014. However, according to the U.S. government’s GPS Web page, “L2C should continue to be considered pre-operational and should be employed at the user’s own risk.” Once the full constellation of GPS satellites transmits the L2C signal, “The U.S. government encourages all users of codeless/semi-codeless GPS technology to plan on using the modernized civil signals by December 31, 2020, as P(Y) may change after that date.” L5 Signals After Block IIR-M, the next generation of satellites, Block IIF, offers a third civilian signal on another frequency band, the L5 band (1176.45 MHz). As opposed to the L2 band, this radio band is reserved exclusively for aviation safety services, and, as such, is expected to be favoured by civilian users over L2 in the future. The first Block IIF satellite was launched in 2010, and, as of July 2015, 8 Block IIF satellites are in operation. The L5 band will be entirely used for civilian signals and free of military signals. Thus, a more powerful signal, with higher bandwidth, when compared with L1 and L2, is transmitted in L5. The main advantages of the L5 signal are: the possibility to track weaker signals through a longer code sequence and better cross-correlations between codes. Additionally, the transmitted power is higher. better immunity to interference with its greater bandwidth, and thus greater spectrum spreading. ionospheric effect attenuation using an L1/L5 combination, similar to the L1/L2 combination. instantaneous or near-instantaneous ambiguity resolution for quicker fixed solution convergence given the use of a triple frequency (L1/L2/L5) signal combination. For a full L5 transmitting satellite constellation, we will have to be more patient than with the L2C full constellation. Whereas the latter is expected by 2020, the former will take several additional years. L1C Signal Compared with modern signals L2C and L5 in operation, the L1 C/A signal is less performant. It is necessary to upgrade it to be on par with the modernized signals. This is where the next generation of GPS satellites, Block III, comes into play. This block of satellites will include another modernized civil signal, the L1C, which will eventually replace the legacy L1 C/A signal. The characteristics of the L1C signal are similar with those of L2C and L5. The design and manufacturing of some of Block III satellites have already been completed, but no launch has taken place yet. First launch is expected during 2016. However, given the current pace of satellite replacement (which can change depending on economic and political unforeseen circumstances), a complete GPS constellation with L1C signals should take at least another decade. The Bottom Line Since the beginning of the new millennium, GPS professionals have impatiently been waiting for all new GPS signals to be fully deployed. Although it will not magically resolve all our current woes such as performance degradation in obstructed areas or long convergence time for a fixed solution, I believe that GPS modernization looks very promising. Legend: Spectrum Use of Legacy and New GPS Signals. Legacy signals: L1 C/A, L1 P(Y) and L2 P(Y). Note that the civilian signal uses the I (in-phase) component and the military signals use the Q (quadrature) component so that they can share the same band. New signals since Block IIR-M: L2C and since Block IIF: L5. Note that there are also new military signals (M) in L1 and L2. Also note that the L5 signal uses both components, I and Q. New signal since Block III: L1C. Note that this signal uses a type of modulation (BOC) that enables it to share the band and in-phase/quadrature component with legacy and military signals.
-
Link: http://effigis.com/obtaining-millimetre-precision-using-satellite-sensors-science-or-fiction/ Precision is a rather sensitive subject in geomatics: talk about it to a surveyor or a GIS specialist, and you better be armed with the right arguments to convince him to change his measuring devices! It’s true that it does not easily spring to mind that the images captured by Earth observation satellites, nowadays with submetric spatial resolution at best, can produce displacement measurements with a precision measured in centimetres or millimetres, as the experts claim. But what exactly does this involve? Let’s first look at optical sensors such as Pléiades (spatial resolution of 0.5 m) and the precision that can be obtained with this type of images. For example, in the case of an open-pit mining site, two digital elevation models (DEM) generated with photogrammetric techniques using stereoscopic pairs acquired on different dates can be compared with determine the location of changes in elevation corresponding to the extraction or piling of rocky material. It is generally accepted that the DEMs produced using Pléiades images, to use this example, have a precision in the order of decimetres. But this is far from being to the centimetre, not to mention the millimetre. For this reason, we must look at radar technology and a very specific way of processing these data. This involves technology known as “differential radar interferometry”, which uses radar images (at least two) taken on a given study site. This technology makes use of synthetic aperture radar (SAR), which can operate at different wavelengths, i.e., at 6 cm for the C-band of the Canadian satellite RADARSAT-2, or at 3 cm for the X-band of the TerraSAR-X and COSMO-SkyMed satellites, to name but a few. In addition to their use for studying the distribution of sea ice, the extent of flooding or even mapping wetlands, these SAR images may indeed be used to measure vertical displacements of the Earth’s surface to the centimetre or millimetre. And how is this possible? It is important to first know that SAR works by transmitting pulses toward the Earth’s surface and recording the portion which is reflected back to the sensor. To be more specific, SAR records the time difference between the transmitted pulse (short sinusoidal signal of centimetric wavelength) and the reception of the reflected energy, its intensity and its phase. In the case of mapping sea ice, floods and wetlands, intensity is of greatest interest. This intensity is based on several factors, including geometry, roughness and humidity of the target on the ground. In interferometry, it is mostly the phase that interests us. Indeed, the phase difference between the two acquisitions taken from different, but close orbits, known as an “interferogram”, provides for each pixel information on topography or ground deformation or displacement. In the latter case, we speak of differential interferometry (DInSAR), since we are interested in the movement that took place between the two consecutive images taken during a given time interval. Legend: Diagram illustrating the principle of differential interferometry Simply stated, since the measurements obtained using interferometry correspond to the difference in the phase between two SAR acquisitions, precision is to a fraction of the wavelength of the sensor used. And this is where it becomes a bit complicated… the interferometric phase is the result of several factors in addition to the actual movement of the target on the ground. These factors are related to, among other things, the geometry of the satellite during the two passages (easily modelled using orbital data), the topography (may be calculated using a DEM), changes in the atmosphere (noise that can be difficult to eliminate) and changes in the characteristics of the target (such as humidity on the Earth’s surface, vegetation conditions or snow cover). Therefore, one must be able to take into account all these factors, which may cause a change in phase during the time between the two images that were used to produce the interferogram. Changes in the characteristics of the target on the ground between the two acquisitions, known as “temporal decorrelation”, is one of the most important factors limiting the application of radar interferometry, since it involves a loss in information regarding the phase between the corresponding pixels of the image pair. In cases like this, we would say that the coherence is low. This decorrelation is a result of the changes related to, for example, the growth or deterioration of the vegetation between the two dates. Therefore, it is important to work with stable targets on the ground (in terms of the radar echo and not in terms of movement), in other words, objects with high coherence, such as buildings, infrastructures, rock outcrops, etc. As for the noise caused by atmospheric effects, it may be reduced by filtering or using multiple interferograms, i.e., several images acquired on successive dates. In fact, it is customary to carry out several acquisitions in order to detect low-amplitude surface movements (or ground deformation) over various time periods. The multiple interferograms are processed as a temporal series whose trend allows us to estimate the rate (cm/unit of time) of surface deformation or displacement. With the various radar satellites currently in operation, the revisit frequency on a given site may vary between 4 and 60 days. Legend: Differential interferogram generated using a pair of RADARSAT-2 images Although it is impossible in certain cases to calculate ground deformation or motion from a given interferogram, primarily because of temporal decorrelation (for example, due to the presence of snow on one of the images), there are many other cases where multiple interferograms are available for which centrimetric or even millimetric precision can be obtained. This is how we can monitor volcanoes on a regular basis by detecting ground deformation related to heaving: relatively sustained movements may be indicators of an imminent volcanic eruption. Similarly, we can use radar interferometry to monitor land subsidence due to thawing permafrost in arctic and sub-arctic regions, or, when conditions allow, due to the extraction of natural gas or oil. It can also be used to monitor the stability of a retaining structure, such as a hydro-electric dam, electric transmission towers or other infrastructures. Radar interferometry has proven to be an especially valuable tool for obtaining information from remote (or dangerous) sites where other types of measurements would be very expensive. In my opinion, this state-of-the-art technology certainly has a place among the precision measurement tools used in geomatics.
-
- 1
-
-
- Satellite Imagery
- Sensors
- (and 5 more)